Harnessing the power of light for healthcare
Light has always played a central role in healthcare, enabling a wide range of tools and techniques for diagnosing and treating disease. Nick Stone from the University of Exeter is a pioneer in this field, working with technologies ranging from laser-based cancer therapies to innovative spectroscopy-based diagnostics. Stone was recently awarded the Institute of Physics’ Rosalind Franklin Medal and Prize for developing novel Raman spectroscopic tools for rapid in vivo cancer diagnosis and monitoring. Physics World’s Tami Freeman spoke with Stone about his latest research.
What is Raman spectroscopy and how does it work?
Think about how we see the sky. It is blue due to elastic (specifically Rayleigh) scattering – when an incident photon scatters off a particle without losing any energy. But in about one in a million events, photons interacting with molecules in the atmosphere will be inelastically scattered. This changes the energy of the photon as some of it is taken by the molecule to make it vibrate.
If you shine laser light on a molecule and cause it to vibrate, the photon that is scattered from that molecule will be shifted in energy by a specific amount relating to the molecule’s vibrational mode. Measuring the wavelength of this inelastically scattered light reveals which molecule it was scattered from. This is Raman spectroscopy.
Because most of the time we’re working at room or body temperatures, most of what we observe is Stokes Raman scattering, in which the laser photons lose energy to the molecules. But if a molecule is already vibrating in an excited state (at higher temperature), it can give up energy and shift the laser photon to a higher energy. This anti-Stokes spectrum is much weaker, but can be very useful – as I’ll come back to later.
How are you using Raman spectroscopy for cancer diagnosis?
A cell in the body is basically a nucleus: one set of molecules, surrounded by the cytoplasm: another set of molecules. These molecules change subtlety depending on the phenotype [set of observable characteristics] of the particular cell. If you have a genetic mutation, which is what drives cancer, the cell tends to change its relative expression of proteins, nucleic acids, glycogen and so on.
We can probe these molecules with light, and therefore determine their molecular composition. Cancer diagnostics involves identifying minute changes between the different compositions. Most of our work has been in tissues, but it can also be done in biofluids such as tears, blood plasma or sweat. You build up a molecular fingerprint of the tissue or cell of interest, and then you can compare those fingerprints to identify the disease.
We tend to perform measurements under a microscope and, because Raman scattering is a relatively weak effect, this requires good optical systems. We’re trying to use a single wavelength of light to probe molecules of interest and look for wavelengths that are shifted from that of the laser illumination. Technology improvements have provided holographic filters that remove the incident laser wavelength readily, and less complex systems that enable rapid measurements.
Raman spectroscopy can classify tissue samples removed in cancer surgery, for example. But can you use it to detect cancer without having to remove tissue from the patient?
Absolutely, we’ve developed probes that fit inside an endoscope for diagnosing oesophageal cancer.
Earlier in my career I worked on photodynamic therapy. We would look inside the oesophagus with an endoscope to find disease, then give the patient a phototoxic drug that would target the diseased cells. Shining light on the drug causes it to generate singlet oxygen that kills the cancer cells. But I realized that the light we were using could also be used for diagnosis.
Currently, to find this invisible disease, you have to take many, many biopsies. But our in vivo probes allow us to measure the molecular composition of the oesophageal lining using Raman spectroscopy, to be and determine where to take biopsies from. Oesophageal cancer has a really bad outcome once it’s diagnosed symptomatically, but if you can find the disease early you can deliver effective treatments. That’s what we’re trying to do.
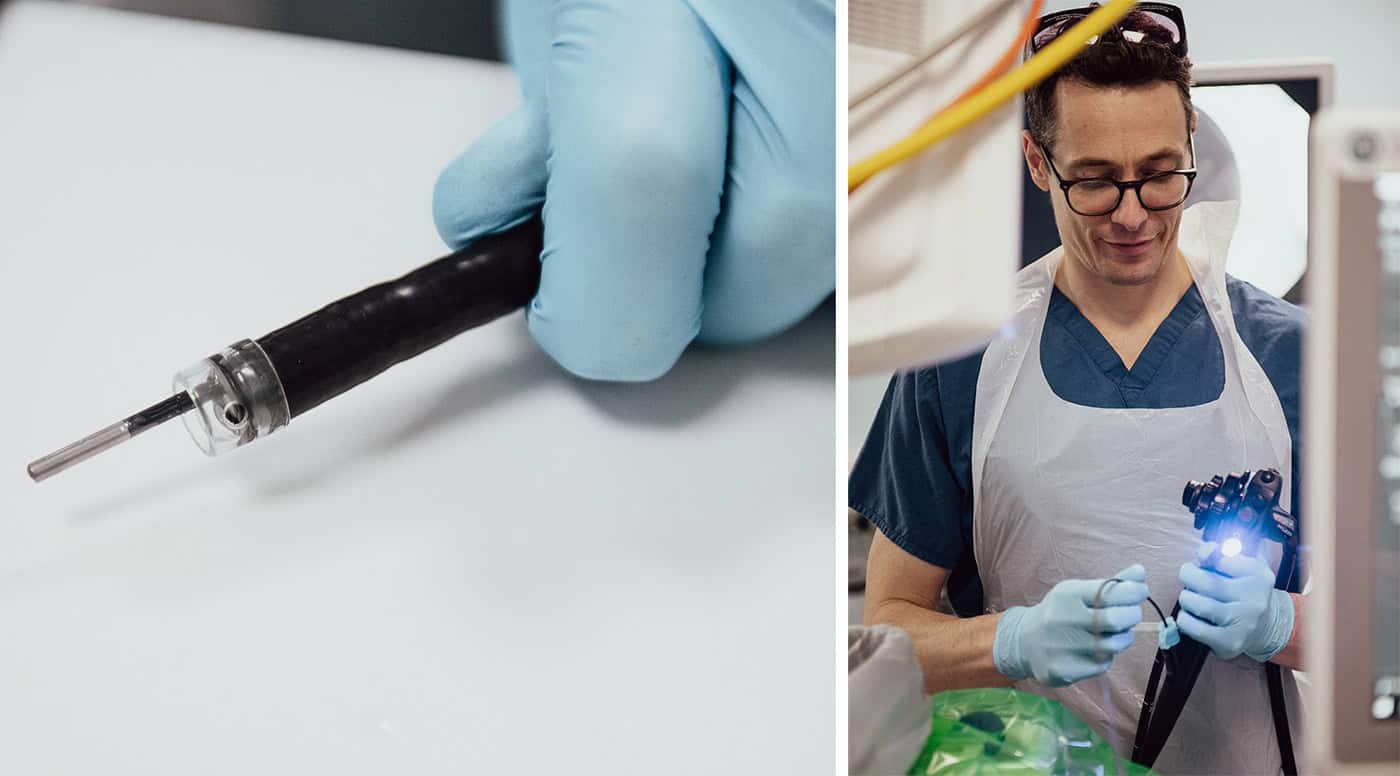
The very weak Raman signal, however, causes problems. With a microscope, we can use advanced filters to remove the incident laser wavelength. But sending light down an optical fibre generates unwanted signal, and we also need to remove elastically scattered light from the oesophagus. So we had to put a filter on the end of this tiny 2 mm fibre probe. In addition, we don’t want to collect photons that have travelled a long way through the body, so we needed a confocal system. We built a really complex probe, working in collaboration with John Day at the University of Bristol – it took a long time to optimize the optics and the engineering.
Are there options for diagnosing cancer in places that can’t be accessed via an endoscope?
Yes, we have also developed a smart needle probe that’s currently in trials. We are using this to detect lymphomas – the primary cancer in lymph nodes – in the head and neck, under the armpit and in the groin.
If somebody comes forward with lumps in these areas, they usually have a swollen lymph node, which shows that something is wrong. Most often it’s following an infection and the node hasn’t gone back down in size.
This situation usually requires surgical removal of the node to decide whether cancer is present or not. Instead, we can just insert our needle probe and send light in. By examining the scattered light and measuring its fingerprint we can identify if it’s lymphoma. Indeed, we can actually see what type of cancer it is and where it has come from.
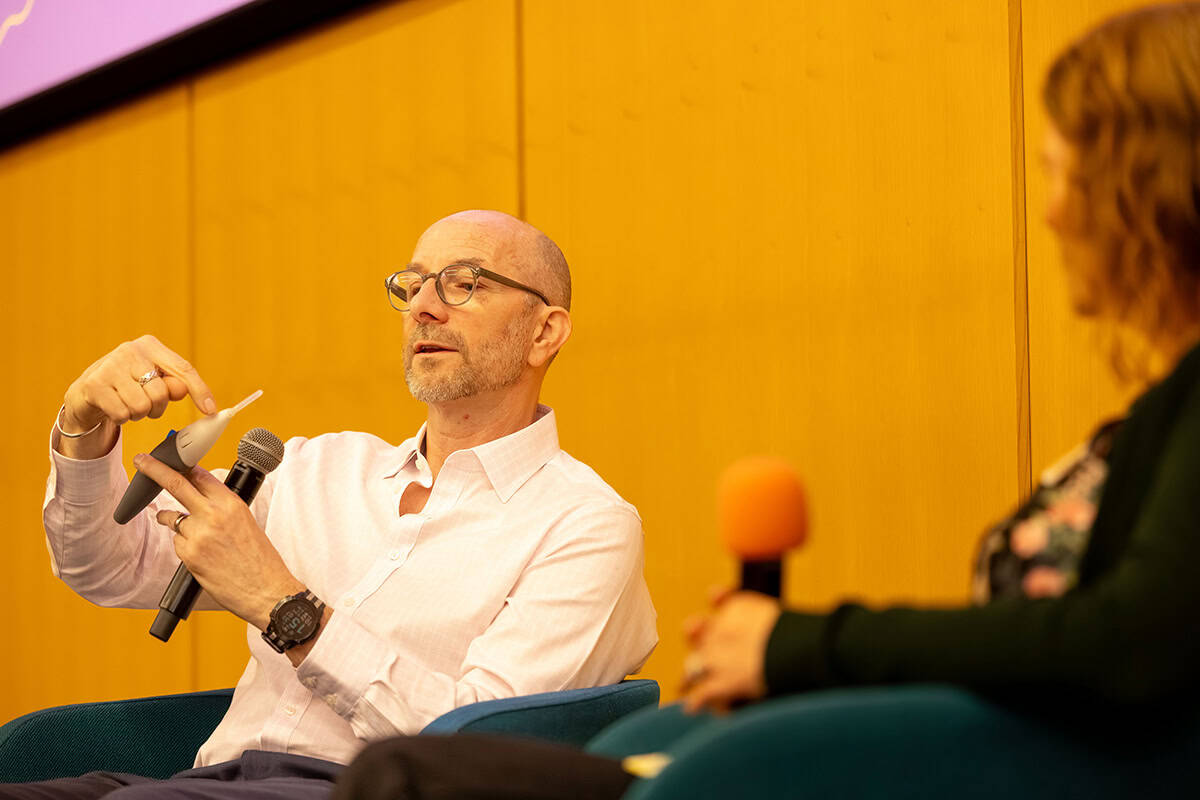
Currently, the prototype probe is quite bulky because we are trying to make it low in cost. It has to have a disposable tip, so we can use a new needle each time, and the filters and optics are all in the handpiece.
Are you working on any other projects at the moment?
As people don’t particularly want a needle stuck in them, we are now trying to understand where the photons travel if you just illuminate the body. Red and near-infrared light travel a long way through the body, so we can use near-infrared light to probe photons that have travelled many, many centimetres.
We are doing a study looking at calcifications in a very early breast cancer called ductal carcinoma in situ (DCIS) – it’s a Cancer Research UK Grand Challenge called DCIS PRECISION, and we are just moving on to the in vivo phase.
Calcifications aren’t necessarily a sign of breast cancer – they are mostly benign; but in patients with DCIS, the composition of the calcifications can show how their condition will progress. Mammographic screening is incredibly good at picking up breast cancer, but it’s also incredibly good at detecting calcifications that are not necessarily breast cancer yet. The problem is how to treat these patients, so our aim is to determine whether the calcifications are completely fine or if they require biopsy.
We are using Raman spectroscopy to understand the composition of these calcifications, which are different in patients who are likely to progress onto invasive disease. We can do this in biopsies under a microscope and are now trying to see whether it works using transillumination, where we send near-infrared light through the breast. We could use this to significantly reduce the number of biopsies, or monitor individuals with DCIS over many years.
Light can also be harnessed to treat disease, for example using photodynamic therapy as you mentioned earlier. Another approach is nanoparticle-based photothermal therapy, how does this work?
This is an area I’m really excited about. Nanoscale gold can enhance Raman signals by many orders of magnitude – it’s called surface-enhanced Raman spectroscopy. We can also “label” these nanoparticles by adding functional molecules to their surfaces. We’ve used unlabelled gold nanoparticles to enhance signals from the body and labelled gold to find things.
During that process, we also realized that we can use gold to provide heat. If you shine light on gold at its resonant frequency, it will heat the gold up and can cause cell death. You could easily blow holes in people with a big enough laser and lots of nanoparticles – but we want to do is more subtle. We’re decorating the tiny gold nanoparticles with a label that will tell us their temperature.
By measuring the ratio between Stokes and anti-Stokes scattering signals (which are enhanced by the gold nanoparticles), we can measure the temperature of the gold when it is in the tumour. Then, using light, we can keep the temperature at a suitable level for treatment to optimize the outcome for the patient.
Ideally, we want to use 100 nm gold particles, but that is not something you can simply excrete through the kidneys. So we’ve spent the last five years trying to create nanoconstructs made from 5 nm gold particles that replicate the properties of 100 nm gold, but can be excreted. We haven’t demonstrated this excretion yet, but that’s the process we’re looking at.
This research is part of a project to combine diagnosis and heat treatment into one nanoparticle system – if the Raman spectra indicate cancer, you could then apply light to the nanoparticle to heat and destroy the tumour cells. Can you tell us more about this?
We’ve just completed a five-year programme called Raman Nanotheranostics. The aim is to label our nanoparticles with appropriate antibodies that will help the nanoparticles target different cancer types. This could provide signals that tell us what is or is not present and help decide how to treat the patient.
We have demonstrated the ability to perform treatments in preclinical models, control the temperature and direct the nanoparticles. We haven’t yet achieved a multiplexed approach with all the labels and antibodies that we want. But this is a key step forward and something we’re going to pursue further.
We are also trying to put labels on the gold that will enable us to measure and monitor treatment outcomes. We can use molecules that change in response to pH, or the reactive oxygen species that are present, or other factors. If you want personalized medicine, you need ways to see how the patient reacts to the treatment, how their immune system responds. There’s a whole range of things that will enable us to go beyond just diagnosis and therapy, to actually monitor the treatment and potentially apply a boost if the gold is still there.
Looking to the future, what do you see as the most promising applications of light within healthcare?
Light has always been used for diagnosis: “you look yellow, you’ve got something wrong with your liver”; “you’ve got blue-tinged lips, you must have oxygen depletion”. But it’s getting more and more advanced. I think what’s most encouraging is our ability to measure molecular changes that potentially reveal future outcomes of patients, and individualization of the patient pathway.
But the real breakthrough is what’s on our wrists. We are all walking around with devices that shine light in us – to measure heartbeat, blood oxygenation and so on. There are already Raman spectrometers that sort of size. They’re not good enough for biological measurements yet, but it doesn’t take much of a technology step forward.
I could one day have a chip implanted in my wrist that could do all the things the gold nanoconstructs might do, and my watch could read it out. And this is just Raman – there are a whole host of approaches, such as photoacoustic imaging or optical coherence tomography. Combining different techniques together could provide greater understanding in a much less invasive way than many traditional medical methods. Light will always play a really important role in healthcare.
- This article is based on a session at the Institute of Physics’ Celebration of Physics in April 2025.
The post Harnessing the power of light for healthcare appeared first on Physics World.