Photonics West shines a light on optical innovation
SPIE Photonics West, the world’s largest photonics technologies event, takes place in San Francisco, California, from 25 to 30 January. Showcasing cutting-edge research in lasers, biomedical optics, biophotonics, quantum technologies, optoelectronics and more, Photonics West features leaders in the field discussing the industry’s challenges and breakthroughs, and sharing their research and visions of the future.
As well as 100 technical conferences with over 5000 presentations, the event brings together several world-class exhibitions, kicking off on 25 January with the BiOS Expo, the world’s largest biomedical optics and biophotonics exhibition.
The main Photonics West Exhibition starts on 28 January. Hosting more than 1200 companies, the event highlights the latest developments in laser technologies, optoelectronics, photonic components, materials and devices, and system support. The newest and fastest growing expo, Quantum West, showcases photonics as an enabling technology for a quantum future. Finally, the co-located AR | VR | MR exhibition features the latest extended reality hardware and systems. Here are some of the innovative products on show at this year’s event.
HydraHarp 500: a new era in time-correlated single-photon counting
Photonics West sees PicoQuant introduce its newest generation of event timer and time-correlated single-photon counting (TCSPC) unit – the HydraHarp 500. Setting a new standard in speed, precision and flexibility, the TCSPC unit is freely scalable with up to 16 independent channels and a common sync channel, which can also serve as an additional detection channel if no sync is required.
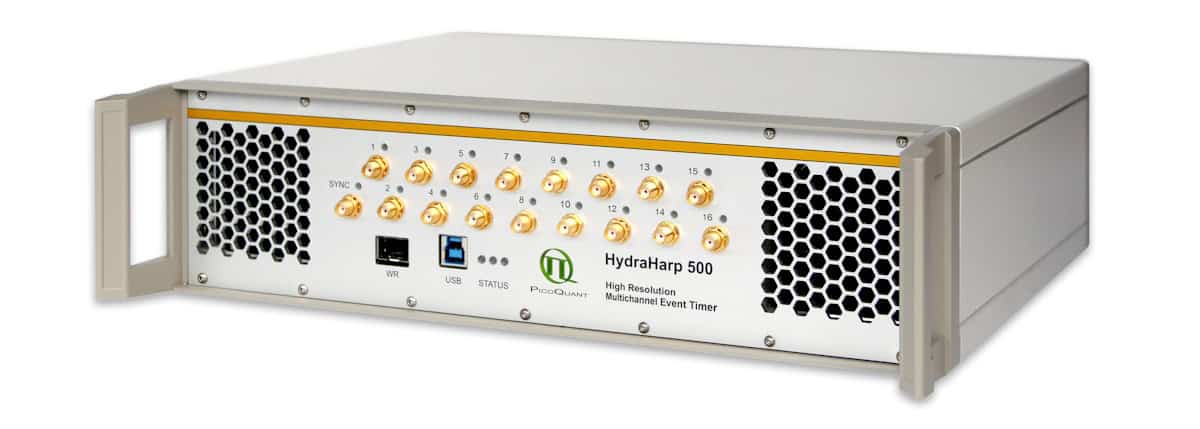
At the core of the HydraHarp 500 is its outstanding timing precision and accuracy, enabling precise photon timing measurements at exceptionally high data rates, even in demanding applications.
In addition to the scalable channel configuration, the HydraHarp 500 offers flexible trigger options to support a wide range of detectors, from single-photon avalanche diodes to superconducting nanowire single-photon detectors. Seamless integration is ensured through versatile interfaces such as USB 3.0 or an external FPGA interface for data transfer, while White Rabbit synchronization allows precise cross-device coordination for distributed setups.
The HydraHarp 500 is engineered for high-throughput applications, making it ideal for rapid, large-volume data acquisition. It offers 16+1 fully independent channels for true simultaneous multi-channel data recording and efficient data transfer via USB or the dedicated FPGA interface. Additionally, the HydraHarp 500 boasts industry-leading, extremely low dead-time per channel and no dead-time across channels, ensuring comprehensive datasets for precise statistical analysis.
Step into the future of photonics and quantum research with the HydraHarp 500. Whether it’s achieving precise photon correlation measurements, ensuring reproducible results or integrating advanced setups, the HydraHarp 500 redefines what’s possible – offering
precision, flexibility and efficiency combined with reliability and seamless integration to
achieve breakthrough results.
For more information, visit www.picoquant.com or contact us at info@picoquant.com.
- Meet PicoQuant at BiOS booth #8511 and Photonics West booth #3511.
SmarAct: shaping the future of precision
SmarAct is set to make waves at the upcoming SPIE Photonics West, the world’s leading exhibition for photonics, biomedical optics and laser technologies, and the parallel BiOS trade fair. SmarAct will showcase a portfolio of cutting-edge solutions designed to redefine precision and performance across a wide range of applications.
At Photonics West, SmarAct will unveil its latest innovations, as well as its well-established and appreciated iris diaphragms and optomechanical systems. All of the highlighted technologies exemplify SmarAct’s commitment to enabling superior control in optical setups, a critical requirement for research and industrial environments.
Attendees can also experience the unparalleled capabilities of electromagnetic positioners and SmarPod systems. With their hexapod-like design, these systems offer nanometre-scale precision and flexibility, making them indispensable tools for complex alignment tasks in photonics and beyond.
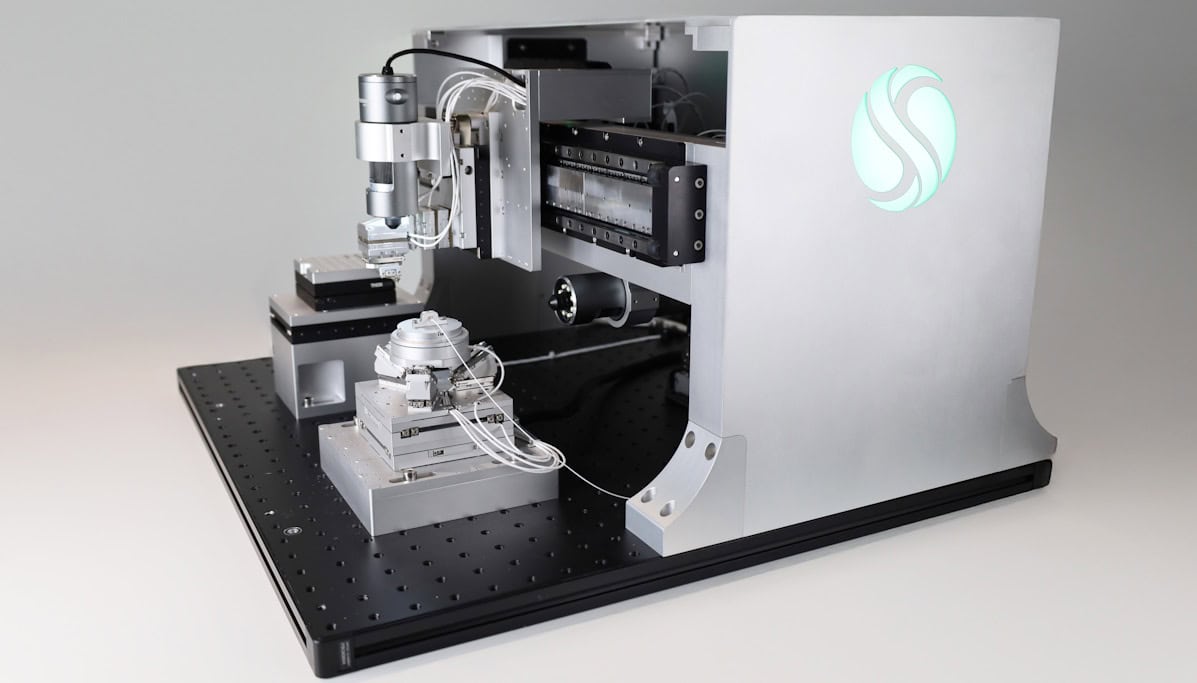
One major highlight is SmarAct’s debut of a 3D pick-and-place system designed for handling optical fibres. This state-of-the-art solution integrates precision and flexibility, offering a glimpse into the future of fibre alignment and assembly. Complementing this is a sophisticated gantry system for microassembly of optical components. Designed to handle large travel ranges with remarkable accuracy, this system meets the growing demand for precision in the assembly of intricate optical technologies. It combines the best of SmarAct’s drive technologies, such as fast (up to 1 m/s) and durable electromagnetic positioners and scanner stages based on piezo-driven mechanical flexures with maximum scanning speed and minimum scanning error.
Simultaneously, at the BiOS trade fair SmarAct will spotlight its new electromagnetic microscopy stage, a breakthrough specifically tailored for life sciences applications. This advanced stage delivers exceptional stability and adaptability, enabling researchers to push the boundaries of imaging and experimental precision. This innovation underscores SmarAct’s dedication to addressing the unique challenges faced by the biomedical and life sciences sectors, as well as bioprinting and tissue engineering companies.
Throughout the event, SmarAct’s experts will demonstrate these solutions in action, offering visitors an interactive and hands-on understanding of how these technologies can meet their specific needs. Visit SmarAct’s booths to engage with experts and discover how SmarAct solutions can empower your projects.
Whether you’re advancing research in semiconductors, developing next-generation photonic devices or pioneering breakthroughs in life sciences, SmarAct’s solutions are tailored to help you achieve your goals with unmatched precision and reliability.
- For more information, visit SmarAct at Photonics West booth #3439, BiOS booth #8439 or explore SmarAct’s application examples here.
Precision positioning systems enable diverse applications
For 25 years Mad City Labs has provided precision instrumentation for research and industry – including nanopositioning systems, micropositioners, microscope stages and platforms, single-molecule microscopes, atomic-force microscopes (AFMs) and customized solutions.
The company’s newest micropositioning system – the MMP-UHV50 – is a modular, linear micropositioner designed for ultrahigh-vacuum (UHV) environments. Constructed entirely from UHV-compatible materials and carefully designed to eliminate sources of virtual leaks, the MMP-UHV50 offers 50 mm travel range with 190 nm step size and a maximum vertical payload of 2 kg.
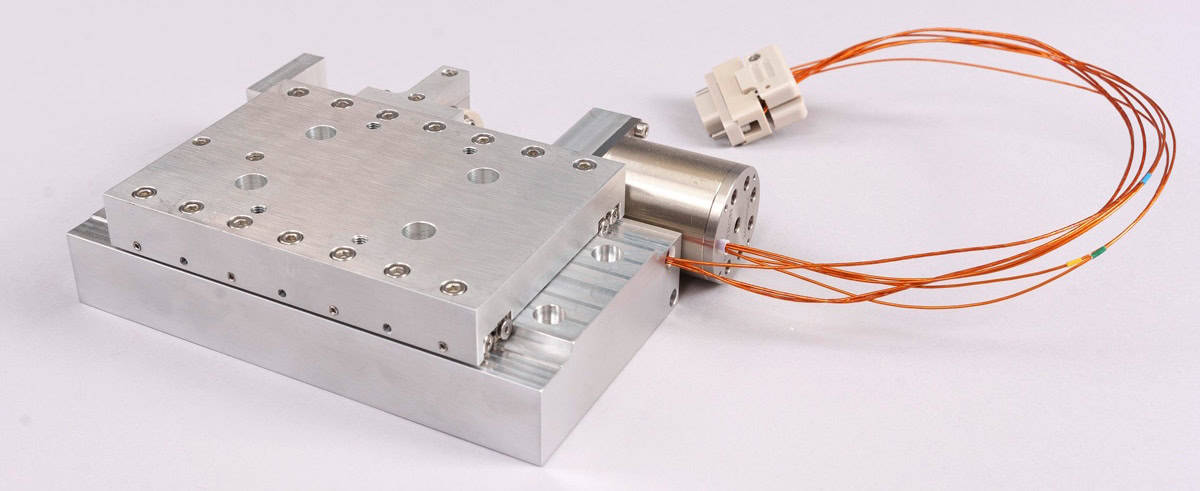
Uniquely, the MMP-UHV50 incorporates a zero-power feature when not in motion, to minimize heating and drift. Safety features include limit switches and overheat protection – critical features when operating in vacuum environments. The system includes the Micro-Drive-UHV digital electronic controller, supplied with LabVIEW-based software and compatible with user-written software via the supplied DLL file (for example, Python, Matlab or C++).
Other products from Mad City Labs include piezo nanopositioners featuring the company’s proprietary PicoQ sensors, which provide ultralow noise and excellent stability to yield sub-nanometre resolution. These high-performance sensors enable motion control down to the single picometre level.
For scanning probe microscopy, Mad City Labs’s nanopositioning systems provide true decoupled motion with virtually undetectable out-of-plane movement, while their precision and stability yields high positioning performance and control. The company offers both an optical deflection AFM – the MadAFM, a multimodal sample scanning AFM in a compact, tabletop design and designed for simple installation – plus resonant probe AFM models.
The resonant probe products include the company’s AFM controllers, MadPLL and QS-PLL, which enable users to build their own flexibly configured AFMs using Mad City Labs’ micro- and nanopositioners. All AFM instruments are ideal for material characterization, but the resonant probe AFMs are uniquely suitable for quantum sensing and nano-magnetometry applications.
Mad City Labs also offers standalone micropositioning products, including optical microscope stages, compact positioners for photonics and the Mad-Deck XYZ stage platform, all of which employ proprietary intelligent control to optimize stability and precision. They are also compatible with the high-resolution nanopositioning systems, enabling motion control across micro-to-picometre length scales.
Finally, for high-end microscopy applications, the RM21 single-molecule microscope, featuring the unique MicroMirror TIRF system, offers multi-colour total internal-reflection fluorescence microscopy with an excellent signal-to-noise ratio and efficient data collection, along with an array of options to support multiple single-molecule techniques.
Our product portfolio, coupled with our expertise in custom design and manufacturing, ensures that we are able to provide solutions for nanoscale motion for diverse applications such as astronomy, photonics, metrology and quantum sensing.
- Learn more at BiOS booth #8525 and Photonics West booth #3525.
The post Photonics West shines a light on optical innovation appeared first on Physics World.