News - Super Mario Kart SNES débarque sur Nintendo Music
Chaque semaine, un nouveau jeu est mis à l'honneur sur NIntendo Music. Et cette fois il ne s'agit pas de n'importe lequel. Découvrez qui est l'heureux élu dans cet article.
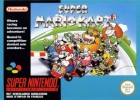
Présenté durant ce Developer_Direct de Xbox ce mercredi 23 janvier, Clair Obscur: Expedition 33 dévoile de nouveaux éléments de son univers, ainsi que le jour de sa sortie. Une nouvelle très attendue par la communauté des amateurs de RPG à la recherche d’un vent de fraicheur.
Nous le savons désormais, Clair Obscur: Expedition 33, le prochain jeu de jeu du studio français Sandfall Interactive, annonce une date de sortie prévue le jeudi 24 avril 2025. Il sera disponible également sur le Game Pass le jour même.
Durant cette présentation, la petite équipe de Montpellier aborde de nouveaux éléments propre à cet univers et revient sur les points les plus importants du jeu pour eux : la réinvention d’un système de combat qui allie le fun et l’exigence. Et un univers immersif qui donne ce sentiment d’exploration que devrait avoir une véritable expédition.
Déjà mis en avant lors des précédents trailers, Clair Obscur: Expedition 33 propose aux joueurs une mécanique dynamique qui vous permet de contrer et d’esquiver les attaques ennemies et de renforcer les vôtres en appuyant sur les bonnes combinaisons de toucher au bon moment. Tout cela alors que le jeu est en tour-par-tour. Un gameplay intéressant qui permet de se préparer avant d’entamer des séquences intenses après avoir choisi ses stratégies. Cette nouvelle présentation met en avant les mécaniques propres aux personnages. Chacun aura un arbre de compétences en lien avec son rôle, ses thèmes et son caractère.
Les développeurs ont déjà prévu une beaucoup de combinaisons possibles à partir des capacités des personnages, mais les possibilités deviennent gargantuesques avec l’ajout des « Pictos », des améliorations qui s’acquièrent avec les combats pour devenir des bonus passifs qui pourront être personnalisés pour chacun des personnages. À l’instar de beaucoup d’anciens RPGs, l’équipe sait que vous êtes capables de casser le jeu. Elle espère que vous le ferez et vous donne tous les outils pour trouver vos combinaisons préférées.
De nouveaux personnages, ont été introduits : Monoco et Esquie. Ce sont des habitants de ce monde baroque inspiré par l’art nouveau, des Gestrals. Leurs comportements loufoques détonnent au milieu de cet univers qui semble si sombre au premier abord, mais ils apportent un vent de gaieté et remettent en question la perception que peut avoir les personnages sur ce qui les entoure. Monoco est une sorte de philosophe combatif qui vous servira de guide et aura la capacité de se transformer en certains ennemis affrontés. Esquie est plus doux, c’est un compagnon qui vous servira de monture pour arpenter l’immensité des territoires proposés par Clair Obscur: Expedition 33. Sandfall Interactive en profite également pour dévoiler une mécanique propre aux anciens JRPG, qui semblait abandonnée depuis plusieurs années : l’exploration à travers une carte en 3D représentative ! À la manière des anciens Final Fantasy ou des Golden Sun, il vous sera possible d’arpenter l’univers à pied ou à dos d’Esquie pour en découvrir les secrets, les nouvelles régions et les boss cachés.
Comme le dit cet article sur le Xbox Wire, Claire Obscur: Expedition 33 confirme son amour pour les classiques du RPG en leur rendant hommage, mais c’est celui que donne Sandfall Interactive à la communauté des joueurs qui pourrait faire de lui une référence pour les générations futures.
Clair Obscur: Expedition 33 est prévu pour le 24 avril 2025. Préparez-vous à embarquer pour une nouvelle aventure pleine de magies et de couleurs, qui vous rappellera surement les classiques de votre enfance et vous marquera pour longtemps.
Cet article Clair Obscur: Expedition 33 annonce sa date de sortie durant le Developer_Direct 2025 de Xbox est apparu en premier sur JVFrance.
Après l’annonce officielle de Ninja Gaiden 4, Team Ninja n’est pas venu les mains vides lors du Xbox Developer_Direct puisque les joueurs vont pouvoir patienter avec une surprise disponible dès à présent sur Xbox Series, PC et PS5 , à savoir Ninja Gaiden 2 Black.
Quoi de mieux pour patienter que de découvrir ou re-découvrir un titre culte de la franchise Ninja Gaiden ? C’est en tout cas la proposition de Microsoft en proposant dès maintenant Ninja Gaiden 2 Black sur Xbox Seroes, PS5 et PC. Rappelons tout de même que le titre est dès à présent accessible sans coût supplémentaire pour les abonnes au Xbox Game Pass.
Toutefois, il ne s’agira pas d’un fainéant portage puisque cette nouvelle mouture s’offre le luxe de tourner sous Unreal Engine 5, un moteur graphique désormais connu et reconnu pour cette nouvelle génération de consoles. L’objectif des développeurs étant de trouver un compromis entre équilibre et modernité afin de respecter l’ambiance originale du titre tout en y intégrant des ajouts de Sigma 2 sorti à l’époque sur la génération PS3/Xbox 360. Par ailleurs, sachez que le titre proposera un système de progression des armes ajustée pour se rapprocher davantage de l’œuvre originale que de la version Sigma 2.
Ninja Gaiden 2 Black est désormais disponible au prix de 49,99€ sur Xbox Series, PS5 et PC tandis que les possesseurs d’un abonnement Game Pass pourront en profiter gratuitement.
Cet article Surprise, le remaster de Ninja Gaiden 2 Black sous Unreal Engine 5 est déjà disponible sur Xbox, PC et PS5 est apparu en premier sur JVFrance.
Koei Tecmo et Team Ninja annoncent leur partenariat avec le studio PlatinumGames pour donner vie au nouvel épisode d’une série légendaire de l’Action Aventure : Ninja Gaiden 4. Attendu pour la fin de l’année 2025, il sortira sur tous les supports actuels.
Une longue présentation et beaucoup d’informations, voici ce qu’il aura fallu afin de ramener sous le feu des projecteurs la série Ninja Gaiden après une décennie d’absence. Ninja Gaiden 4 mettra en avant un nouveau personnage principal, Yakumo, qui évolue dans un Tokyo dystopique transformé par le retour du Dark Dragon.
Pas d’inquiétude pour les fans de longue date, Ryu Hayabusa, héros iconique de la série, sera également présent, jouable, et central dans l’histoire. Situé dans un Tokyo sombre et cyberpunk, le jeu adopte une esthétique marquée par la corruption du Dark Dragon, avec des effets visuels renforçant l’atmosphère oppressante du monde de Ninja Gaiden 4.
Ninja Gaiden 4 introduira des mécaniques inédites comme les déplacements sur rails et câbles, ajoutant fluidité et intensité aux phases d’action. Yakumo possède une capacité unique appelée Bloodraven Form, permettant de manipuler le sang pour exécuter des attaques dévastatrices, comme le Bloodbath Kill, idéale pour éliminer rapidement des groupes d’ennemis. Néanmoins, le titre promet de rester fidèle à l’ADN de la série, prenant pour inspiration le style développé dans Ninja Gaiden 2. Il conservera une difficulté exigeante et un gameplay conçu pour récompenser la maîtrise des différentes mécaniques.
Si le jeu a été présenté longuement ce soir, avec notamment plusieurs phases de gameplay et pas mal d’informations au sujet de ses inspirations et du monde qui nous attend, la date de sortie exacte n’a pas encore été dévoilée. Le jeu sortira à l’automne 2024, sans plus de détails à l’heure actuelle. Attendons-nous à voir débarquer la célèbre licence sur nos plateformes de jeu dès le mois d’octobre
Ninja Gaiden 4 est donc prévu pour la fin d’année et sera disponible sur PC, Xbox Series X | S et PS5. De plus, le jeu sera inclus dans le Xbox Game Pass dès son lancement.
Cet article Ninja Gaiden 4 est le jeu mystère du Developer_Direct, prévu pour l’automne 2025 est apparu en premier sur JVFrance.
Nous vous partagions l’information plus tôt dans nos colonnes à la suite d’un leak en provenance de nos confrères de chez Gamekult, et le Developer_Direct a officialisé la chose. DOOM: The Dark Ages sortira en mai sur PC et consoles !
Les fans de la célèbre franchise Doom peuvent marquer leurs calendriers de 2025 car DOOM: The Dark Ages, le nouvel opus mêlant action frénétique et ambiance médiévale, débarquera le 15 mai prochain. Cet épisode nous promet de plonger dans une ère de ténèbres où épées, magie, et démons s’entrechoquent pour une expérience brutale. Sans oublier le fameux bouclier du Doom Slayer
Dans sa nouvelle stratégie afin d’élargir son audience, Microsoft confirme la sortie de DOOM: The Dark Ages aussi sur PS5. Nous savons que le géant américain souhaite abandonner sa logique d’exclusivités aux supports PC et Xbox et porter ses IP vers les machines concurrentes, comme cela fut déjà le cas avec Sea of Thieves ou encore Indiana Jones et le Cercle Ancien encore récemment, dont la sortie sur la console de Sony est prévue pour le Printemps 2025.
DOOM: The Dark Ages sera disponible sur PS5, Xbox Series X | S et PC le 15 mai 2025.
Cet article C’est officiel, DOOM: The Dark Ages sortira au mois de mai 2025 est apparu en premier sur JVFrance.
Annonce Annoncé il y a un peu plus de 6 mois, South of Midnight est le prochain jeu d’aventure du studio Compulsion Game, créateur des titres Contrast et We Happy Few. Assez énigmatique malgré une bande-annonce stylisée et une première prise en mains lors de gros évènements, le titre s’offre enfin plus de détails lors du Xbox Developpeur Direct.
Présenté longuement lors du Xbox Developpeur Direct, South of Midnight nous présente son univers ainsi que son gameplay. De quoi se concentrer davantage sur l’aspect des combats en zone, l’arbre de compétence permettant de débloquer de nombreux sorts plus ou moins rapidement, quelques cinématiques, des environnements fantastiques ainsi que les quelques interactions possibles avec celui-ci. Nous apprenons aussi l’intrigue du titre, se déroulant après un ouragan où l’héroine : Hazel, se voit entraînée dans un monde gothique du Sud et où elle devra tout faire pour sauver sa mère. L’une des particularités graphiques du titre se trouve surtout dans sa manière de gérer la saccade d’image, offrant une idée de stop motion à certains moments, surtout lors d’évènements importants ou alors de cinématiques.
Inspiré par les musiques sud-américaines, nous aurons l’occasion de découvrir une grande variété de sonorités qui berceront l’ensemble de l’aventure. Toutefois, ne vous laissez pas amadouer par ces douces mélodies, car South of Midnight sera une véritable histoire initiatique où esprits et créatures fantastiques se mélangent.
South of Midnight sortira le 8 avril 2025 sur le Game Pass, Xbox Series X/S et PC.
Cet article South of Midgnight : La date de sortie a été dévoilée durant le Developer_Direct est apparu en premier sur JVFrance.
Il arrive enfin après de multiples reports, Assassin’s Creed Shadows devrait bien sortir le 20 mars 2025 sur consoles et PC. Malgré les nombreuses polémiques entourant le titre, et notamment l’un de ses personnages principaux, ce nouvel épisode doit marquer un tournant pour la franchise puisqu’il s’agit du premier titre qui ne sortira pas sur la génération précédente de consoles. Nous avons pu mettre la main sur cette production attendue pendant près de trois heures, et voici nos premières impressions.
Preview réalisée lors d’un événement presse organisé en streaming (via la technologie PARSEC) par l’éditeur
Lors de la diffusion de sa première bande-annonce l’année dernière, Assassin’s Creed Shadows avait largement mis en avant deux protagonistes : Yasuke et Naoe. Si le premier paraissait bien plus lourd et assez peu adapté aux phases de parkour, il avait l’air d’être un combattant hors pair tandis que la seconde protagoniste était plus légère, plus habile, incarnant davantage l’assassin idéal finalement. Ces impressions se sont confirmées lors de notre première prise en main : bien qu’ayant des vies bien différentes et semées d’embûches, nos deux protagonistes se retrouvent pour une cause similaire que nous tairons. Pour la première fois dans l’histoire de la franchise, Assassin’s Creed Shadows nous met dans la peau de deux assassins, proposant ainsi deux approches bien distinctes : l’infiltration et le passage en force. Naoe est le personnage idéal pour l’infiltration, grâce à une facilité de déplacement nullement vue auparavant, incarnant la grâce et la légèreté. Yasuke, quant à lui, est vêtu d’une armure des plus solides. Au combat, c’est une véritable machine de guerre, un tank pour être totalement précis, et chacune de ses frappes inflige des dégâts considérables.
Contrairement à Assassin’s Creed Mirage où il était assez facile de s’en sortir lorsque nous étions face à quelques gardes, Assassin’s Creed Shadows demande de bien réfléchir son approche avant de passer à l’action. En effet, chaque impact reçu est dévastateur, d’autant plus chez Naoe. Ce n’est pas compliqué, avec elle, trois coups reçus et nous étions déjà au sol à recharger une sauvegarde récente. L’IA adverse ne fait pas dans la demi-mesure et c’est une excellente chose. Bien entendu, et à l’instar des précédents épisodes, il faudra faire le choix entre une parade et une esquive en fonction de l’attaque de votre opposant. Les attaques lourdes nécessiteront une esquive tandis qu’une attaque légère demandera une parade. Si votre geste est parfait, vous pourrez comme d’habitude prendre l’ascendant en effectuant un coup critique. Toutefois, nous avons été quelque peu dérangés par le lock manuel qui ne réagit pas en cas d’attaque d’un autre adversaire : un coup à prendre qui n’a pas facilité notre approche des combats. Comme toujours, chaque personnage dispose d’attaques spéciales que nous pouvons choisir depuis le menu approprié afin de se faciliter la vie. Avoir des rations sur soi sera ainsi indispensable pour augmenter ses chances de survie, tandis que l’évaluation du terrain sera nécessaire pour travailler votre approche.
En effet, il sera nécessaire d’utiliser la gachette R2 (nous avons joué avec une manette DualSense), pour identifier tous les ennemis et ainsi faciliter notre infiltration. Un seul ennemi non identifié mettrait en péril une approche discrète. Bien entendu, la vision d’aigle pourra simplifier cette détection, mais il s’agira ici d’une compétence à assigner à vos assassins. Parce que oui, l’arbre de compétences de ce Assassin’s Creed Shadows a l’air colossal et l’acquisition de ces compétences devra être réfléchie. Chaque héros disposera de ses propres compétences séparées en six thèmes. Ainsi, ce ne sont pas moins de 12 arbres de compétences différents que vous pourrez alimenter au cours de votre quête.
À l’instar des précédents épisodes, le Japon sera divisé en plusieurs régions qui auront chacune un niveau d’ennemi. Cela signifie que vous pourrez explorer librement toutes les régions, mais attention : si vous croisez un ennemi dans une région où vous n’avez pas le niveau requis, un seul coup et c’est la mort. La progression s’effectuera donc naturellement en fonction de l’évolution de votre personnage. Pour conclure sur cette partie carte, sachez que le monde proposé semble immense à première vue, mais plutôt bien équilibré avec de belles contrées à explorer. Selon l’éditeur français, la taille de la carte serait proche de celle d’Assassin’s Creed Origins, l’un des meilleurs opus grâce notamment à sa dimension humaine. Enfin, et à l’instar de ce que proposait Assassin’s Creed Valhalla, il sera possible d’améliorer son repaire. Cette fois-ci, une plus grande liberté sera donnée dans la construction des batiments avec la possibilité notamment de choisir les emplacements, d’y ajouter des arbres et autres décorations. Les alliés qui vous aideront en combat pourront être améliorés par ce biais-là. Nous n’avons pas pu y jouer lors de cette preview, mais Ubisoft nous a confirmé que cette fonctionnalité sera bien disponible dès la sortie du titre.
Naoe et Yasuke ont eu tous deux des destins bien différents qui ont fait que leurs routes se sont croisées à un moment donné. Si Naoe est une guerrière animée par la haine et la vengeance, Yasuke, quant à lui, est un esclave de longue date qui a su se libérer des griffes de son maître pour désormais faire régner la paix et la prospérité. Nous avons débuter notre preview par le tout début du jeu qui servait de tutoriel, mais juste avant cela, nous avons pu découvrir le hub Animus. Il s’agit ici d’un nouveau launcher qui regroupe tous les épisodes de la série Assassin’s Creed depuis Origins. Fonctionnant sous forme de frise chronologique, ce hub permet de sélectionner rapidement la mémoire (ou le titre) souhaité et de le lancer directement. Un petit plus sympathique qui permettra une meilleure compréhension dans la chronologie de la saga qui sera disponible sur PC, mais également sur consoles. Au premier lancement de ce nouvel opus, nous avons le droit de sélectionner quelques paramètres : langue des dialogues, langue du texte et difficulté désirée. Nous avons sélectionné le mode normal qui est tout de même le troisième choix sur quatre possibles, ce qui en dit long déjà sur la potentielle difficulté du titre. Enfin, il existe une fonction histoire imposée. Cette dernière retire ainsi tous les choix déterminants et moraux à faire pour se laisser guider par les choix recommandés par les développeurs.
À l’instar de ce que proposait Assassin’s Creed Mirage avec la présence de la langue arabe, Assassin’s Creed Shadows propose aussi un mode immersion pour avoir les dialogues dans la langue d’origine, à savoir le japonais, mais également le portugais. Pourquoi le portugais nous demanderez-vous ? Vous le découvrirez lorsque vous aurez l’occasion de poser les mains sur le jeu. Il n’y a pas à dire, les doublages japonais sont impeccables et l’immersion au pays du soleil levant est réussie. Il en est de même pour la direction artistique et notamment l’arrivée des saisons. En effet, en plus du fonctionnement classique avec le cycle jour-nuit, il sera possible de sélectionner la saison souhaitée avec un changement visuel des plus pertinents. Nous avons hâte de vivre la saison des cerisiers en fleurs dans ce Assassin’s Creed Shadows. Si les dialogues en japonais sont pertinents et bien doublés, nous avons pu assister à de nombreuses cinématiques pendant nos trois heures de jeu. Nous attendons d’avoir le jeu entre nos mains et non en streaming pour donner un avis sur la réalisation globale du titre. En effet, l’absence de versions PS4 et Xbox One devrait permettre à la franchise de franchir un cap graphique non négligeable, et il nous faudra donc attendre la version définitive pour se faire un avis tranché.
Néanmoins, nous n’avons constaté que peu de désagréments lors de cette preview. Notamment dans la gestion des PNJ avec, par exemple, la gestion d’un homme fou qui courait dévêtu en pleine rue. Ce dernier était arrivé à l’emplacement prédéfini, et nous avons dû attendre auprès de lui pendant une dizaine de secondes que son dialogue se termine pour enchaîner correctement. Espérons que ce genre de gêne sera corrigé pour la version définitive du titre. Mis à part ce petit couac, nous avons eu assez peu de bugs durant notre session de jeu. Laissant présager que le report du titre de quelques mois était une bonne décision de la part de l’éditeur français pour éviter un bashing comme lors de la sortie de Star Wars Outlaws, bien que ce dernier était tout de même assez excessif. Si nous avons uniquement parlé de la quête principale fonctionnant sur la même lignée que les derniers opus, il existera évidemment de nombreuses activités annexes. Chaque personnage aura le droit à des quêtes secondaires spécifiques, mais nous n’avons pu y jouer durant notre session. Bien entendu, les points de synchronisation seront toujours de la partie et il sera possible de visualiser les centres d’intérêts aux alentours et de le sélectionner rapidement pour afficher la route à suivre.
En trois heures de jeu, nous avons pu avoir un aperçu de la vaste région proposée par Assassin’s Creed Shadows, mais surtout une logique de gameplay qui ravira les fans de la franchise. Bien que le jeu proposera deux approches totalement différentes via des personnages principaux diamétralement opposés, il vous faudra sélectionner le bon protagoniste pour vous en sortir le mieux possible. On y retrouve, comme toujours, un enchaînement de missions afin de démasquer les templiers et une partie RPG toujours d’actualité, mais qui devrait rester tout de même assez légère. Si l’ambiance japonaise est réussie et très bien retranscrite via les dialogues ou encore les musiques, il faudra valider la réalisation globale le 20 mars prochain.
Cet article PREVIEW | On a testé Assassin’s Creed Shadows sur PC est apparu en premier sur JVFrance.
Comme convenu, Xbox donne rendez-vous à son audience ce jeudi 23 janvier pour assister au premier Developer_Direct de l’année, un évènement en ligne accessible à tous pour découvrir les prochains titres à destination des consoles Xbox Series, PC à venir dans le Game Pass.
Le rendez-vous instauré par Xbox pour présenter les jeux à venir sur ses différentes plateformes devient une sorte d’habitude, avec un déroulé que l’on connait désormais bien : 4 à 5 jeux mis à l’honneur avec des phases de gameplay et une prise de parole des équipes de développement pour en dire plus sur leurs titres.
Ce soir, Xbox nous présentera 3 jeux déjà annoncés, et très attendus :
On notera qu’un quatrième mystérieux jeu sera de la partie. Certaines rumeurs font écho qu’un nouvel opus de la franchise Devil May Cry, mais sans plus de preuves, il nous semble préférable de ne pas trop accorder de crédit à ces bruits de couloir, quand bien même il serait logique que Capcom planche sur un nouvel opus. En effet, Devil May Cry V soufflera sa 6ème bougie cette année.
Comme à l’accoutumée, Xbox diffusera le direct sur ses réseaux officiels. Vous pourrez donc suivre la diffusion en direct à partir de 19h sur les plateformes suivantes :
À noter que nous vous recommandons de suivre l’évènement sur Twitch si vous le pouvez, il y a parfois un léger décalage sur YouTube.
Le rendez-vous est évidemment pris de notre côté et nous ne manquerons pas de vous partager l’annonce du jeu mystère dans nos colonnes.
Cet article Comment suivre le Xbox Developer_Direct du 23 janvier 2025 est apparu en premier sur JVFrance.
Malgré la mini extension L’Île Fabuleuse prenant place dans le set Puissance Génétique, les joueurs de Pokémon TCG Pocket sont restés sur leur faim avec seulement 82 nouvelles cartes. Néanmoins l’attente ne sera plus très longue puisque Choc Spatio-Temporel, la prochaine extension, sortira à la fin du mois et on connait déjà les premières cartes de l’extension.
Si vous faîtes partie des joueurs qui, en un peu plus d’un mois, ont déjà eu le temps de collectionner toutes les cartes de la mini extension du set A1, nul doute que vous attendez avec impatience la nouvelle véritable extension de Pokémon TCG Pocket. En effet, le booster à thème L’Île Fabuleuse ne proposait que 82 cartes, ce qui n’a pas suffit à contenter les collectionneurs en herbe. Nonobstant, ce booster aura eu le mérite d’apporter quelques cartes qui sont venues changer la meta et proposer quelques alternatives viables en combat.
Bien que l’on s’y attendait, notamment grâce aux nombreuses fuites, l’extension Choc Spatio-Temporel sortira bien à la fin du mois de janvier, et très exactement jeudi prochain, soit le 30 janvier 2025. Les fans de la quatrième génération seront ravis d’apprendre que la région de Sinnoh sera mise à l’honneur ici, puisque des Pokémon tels que Tiplouf, Ouisticram, Tortipouss, Lucario, Dialga, Palkia ou encore Darkrai seront disponibles.
L’extension comportera 2 boosters qui mettront en vedette les légendaires Dialga et Palkia. Malgré cela, il s’agit bien ici d’une nouvelle extension (A2) et non pas d’une mini extension comme pouvait l’être L’Île Fabuleuse.
Puisque le style, ça compte, surtout dans Pokémon TCG Pocket qui met l’accent sur la collection, cette nouvelle extension se verra accompagnée de nouveaux accessoires pour compléter le tout. On y retrouvera de nouveaux portofolios et cadres expo mettant en avant Dialga et Palkia, afin de présenter ses nouvelles trouvailles à la communauté. Reste à voir si des nouvelles pièces, dos de cartes et tapis de jeu seront également de la partie ou s’il faudra attendre de nouveaux évènements en jeu pour agrandir sa collection d’objets.
Pokémon TCG Pocket (ou Le Jeu de Cartes à Collectionner Pokémon Pocket pour les chauvins) est toujours disponible sur smartphones et tablettes Android et iOS.
Cet article Première extension Pokémon TCG Pocket de 2025 : Les infos sur Choc Spatio-Temporel est apparu en premier sur JVFrance.
Petite surprise en ce jeudi 25 janvier 2025 puisque la PS5 reçoit la première mise à jour de son firmware depuis désormais plusieurs semaines. Cependant, ne vous attendez pas à des folies en ce qui concerne les nouvelles fonctionnalités proposées.
Cela faisait un bail que le firmware de la PS5 n’avait été mis à jour par PlayStation et c’est désormais chose faite avec cette première mise à jour de l’année 2025. Nommée toujours très sobrement 25.01-10.60.00, cette dernière n’apporte que quelques corrections d’écrans et une amélioration de la stabilité globale du système. Pour télécharger cette nouvelle mise à jour, comptez environ 1,32 Go à récupérer depuis les serveurs de Sony.
Sans plus tarder, découvrez le changelog complet de cette nouvelle mise à jour :
Cet article La mise à jour système 25.01-10.60.00 est disponible sur PS5 (patch note) est apparu en premier sur JVFrance.
Une célèbre rédaction française a visiblement reçu en avance des informations de Bethesda en vue d’une annonce très prochaine concernant DOOM: The Dark Ages. Malheureusement, il semblerait qu’une information déterminante ait fuité avant sa levée d’embargo : il s’agit ni plus ni moins que de la date de sortie du projet.
Après deux opus de très grande qualité, Bethesda va revenir cette année avec une franchise bien connue et appréciée des joueurs. En effet, DOOM: The Dark Ages devrait sortir cette année et le Xbox Developer_Direct qui se tiendra ce jeudi 23 janvier 2025 apportera une date de sortie bien précise. Toutefois, nos confrères de chez Gamekult ont vraisemblablement diffusé par erreur cette information capitale à cause des flux RSS.
Ainsi, DOOM: The Dark Ages devrait être disponible le 15 mai 2025 sur consoles et PC. Une date de sortie idéale pour profiter d’un nouveau FPS nerveux durant tous les ponts qui se préparent avec une quantité importante de jours fériés.
Pour rappel, DOOM: The Dark Ages sera disponible sur PS5, Xbox Series X|S et PC.
Cet article RUMEUR | DOOM: The Dark Ages – La date de sortie serait connue est apparu en premier sur JVFrance.
Pour les détenteurs d’une Xbox Series, la seule possibilité d’augmenter le stockage de la console se trouvait dans l’achat de petits SSD à venir brancher sur l’arrière de la console, mais réservés seulement pour la Xbox. Désormais, Microsoft proposera une extension plus importante du stockage avec des possibilités de fournir plus que 16 To.
La Xbox Series X et S obtiendront de plus grandes options de stockage via la prise en charge de stockage USB externe. Une information confirmée par les membres du Xbox Insider dans le cadre d’une mise à jour du logiciel système, désormais disponible pour les testeurs publics de la session Alpha Skip-Ahead. Selon les notes de version, il y aura un support pour les clés USB externes de plus de 16 To. Toutefois, une petite ombre au tableau se dessine, celle de devoir formater complètement le disque utilisé pour pouvoir utiliser ce changement. Partagé dans un article du blog Xbox, Microsoft précise que les disques formatés le seront avec plusieurs partitions, l’idée étant d’utiliser tout l’espace disponible pour les jeux et les applications Xbox. Ceux-ci apparaîtront alors sous forme de plusieurs périphériques dans la liste des périphériques de stockage.
Ne précisant pas de limite de taille pour les disques, là où nous sommes actuellement limités à 2 To, il se pourrait que nous puissions utiliser des SSD proposant énormément de stockage, mais aussi des HDD, pratiques pour les plus petits budgets. Malheureusement pour ce dernier, il y aurait certains HDD qui ne refléteraient pas les bonnes informations sur l’espace disponible après formatage. Il est donc conseillé de ne pas formater les lecteurs HDD de plus de 16 To pour l’instant. Il est tout à fait normal de tomber sur des bugs, car, rappelons-le, Microsoft se sert des membres de l’Alpha Skip-Ahead comme des beta-testeurs.
En attendant de pouvoir voir cette extension de stockage pour tous, il est toujours possible d’augmenter l’espace libre de la console via l’achat de SSD adéquats à venir brancher directement sur la console.
Cet article Microsoft annonce la prise en charge d’un stockage externe plus important pour la Xbox est apparu en premier sur JVFrance.
Prochain titre des papas de Life is Strange, Don’t Nod décide de repousser le deuxième chapitre de Lost Records: Bloom & Rage pour pouvoir proposer une meilleure optimisation du titre et un meilleur raffinement.
Suivant l’histoire de quatre amis du secondaire sur deux chronologies différentes, Lost Records: Bloom & Rage est le futur titre du studio Don’t Nod qui réitère l’expérience des jeux d’aventure narrative. Proposés en 2 volets : Bloom et Rage, représentant chacun les deux chronologies, Lost Records: Bloom & Rage se déroulera en premier en 1995 lors d’un été « magique » bizarrement nostalgique, et 27 ans plus tard avec des personnages plus grands devant confronter le secret qu’ils ont longtemps enfoui.
Avec un premier report en l’année dernière, et alors que le premier volet est toujours prévu pour le 18 février prochain, le second se voit légèrement retardé pour une sortie prévue le 15 avril 2025 au lieu du 18 mars. Dans un communiqué détaillant les raisons de ce retard, le studio a déclaré qu’il avait pris la décision afin de « permettre une optimisation supplémentaire et de donner à l’équipe le temps nécessaire pour affiner l’expérience pour les joueurs. »
En combinant les deux épisodes, Lost Records: Bloom & Rage offrira une durée de vie de 10 à 12 heures environ. Pour celles et ceux souhaitant obtenir les deux versions en même temps, une édition adéquate sera proposée à la sortie du second épisode, le 15 avril prochain.
Lost Records: Bloom & Rage Tape 1 sortira donc le 18 février 2025 sur PS5, Xbox Series X/S et PC au prix de 39,99€.
Cet article Le chapitre 2 de Lost Records: Bloom & Rage repoussé d’un mois pour une meilleure optimisation est apparu en premier sur JVFrance.
Electronic Arts a dû revoir ses prévisions financières à la baisse pour l’exercice fiscal en cours, suite aux performances décevantes de deux de ses titres majeurs : Dragon Age: The Veilguard et EA Sports FC 25.
Lancé en octobre, Dragon Age: The Veilguard marquait le retour très attendu de la franchise après une décennie d’absence. Cependant, le jeu n’a attiré qu’environ 1,5 million de joueurs durant le dernier trimestre 2024, soit près de 50 % en dessous des attentes. De son côté, EA Sports FC 25, a également enregistré une baisse de ses performances en fin d’année, alors que les prévisions initiales tablaient sur une légère croissance.
EA a donc dû ajuster ses prévisions financières pour l’exercice se terminant en mars 2025 et prévoit des résultats nets de 7 à 7,15 milliards de dollars, contre 7,5 à 7,8 milliards initialement. Pour le trimestre actuel, les prévisions ont, elles aussi, aussi été revues à la baisse, à 2,21 milliards de dollars.
Difficile d’identifier les causes à effet, l’éditeur avait-il été trop ambitieux, ou bien ses prévisions étaient-elles justes et l’attractivité des titres a-t-elle réellement été en deçà des attentes. EA Sports FC a pâti de son changement de nom depuis que la FIFA a récupéré ses droits. Idem, Dragon Age : The Veilguard a changé de nom en cours de route et les premières annonces parlaient de Dragon Age : DreadWolf. Même si on ne peut pas désigner ces éléments comme étant les raisons principales de cette contre-performance, il est vrai que cela ne facilite pas la compréhension chez les joueurs.
Cet article Dragon Age: The Veilguard serait décevant selon EA est apparu en premier sur JVFrance.
SEGA décide de fédérer un peu plus sa communauté de joueurs. Un service pour les enregistrer tous et dans la passion les lier.
Un nouveau service en ligne est maintenant disponible pour tous les joueurs des titres édités par SEGA et ATLUS, Une manière de lier vos comptes et de profiter de bonus sur les jeux proposés par ces deux géants japonais. En vous inscrivant, vous recevrez les dernières nouvelles sur vos jeux favoris, des annonces d’événements majeurs et des opérations promotionnelles sur les titres de SEGA et ATLUS.
Le service en ligne SEGA Account est en partenariat avec Nintendo, PlayStation, Xbox, Steam et Epic Games, prévu pour une mise en ligne le 17 février 2025.
Actuellement, les seuls bonus proposés sont : un skin exclusif au jeu Like a Dragon: Pirate Yakuza in Hawaii, si vous activez votre compte avant le 7 mars 2025. Ainsi qu’une ribambelle de bonus pour le jeu en ligne Phantasy Star Online 2 New Genesis, comme des gemmes étoiles et animations.
Deux titres uniquement son mis en avant pour ce service, dont l’un d’eux qui semble disponible uniquement pour le marché japonais. Mais comme l’annonce la page du service, il y aura également un système d’archives que l’on pourra consulter pour connaître son historique de jeux, ainsi que d’autres titres qui seront ajoutés.
C’est encore bien maigre comme avantages pour vraiment motiver les joueurs à s’enregistrer encore une fois à un service en ligne. Alors que Sonic est utilisé comme visuel pour promouvoir le service, aucun jeux avec lui en vedette n’est mis en avant. Et mis à part pour le MMO PSO2 NG, cité plus haut, le skin proposé pour le spin-off de Yakuza: Like a Dragon n’est pas non plus assez original et thématique.
Cette annonce est assez discrète, vu son manque de poids pour représenter un réel intérêt. Il peut s’agir d’une première démarche pour tester la fonctionnalité du service avant d’y investir plus de moyens. Il reste cependant assez difficile d’y voir un réel intérêt pour le joueur moyen si le service se contente d’y faire des annonces qui seront relayées par des services plus connus ou de proposer des contenus anecdotiques pour les jeux. Qui sait, peut-être aurons-nous droit à un jeu service estampillé SEGA et ATLUS dans les mois qui suivront ? Il faudra par contre travailler la communication, qui rime mal avec discrétion.
Une annonce bien maigre, mais qui possède du potentiel pour l’avenir de SEGA. On vous encourage à vous y inscrire si vous aimez les jeux de ces éditeurs. Vous pourrez y toujours avoir de belles surprises !
Cet article SEGA lance SEGA Account, un service en ligne qui relie vos comptes avec des bonus pour vos jeux est apparu en premier sur JVFrance.
La suite du RPG médiéval ultra immersif, Kingdom Come : Deliverance II, sera officiellement disponible dans quelques jours. Warhorse Studio dévoile davantage de détails sur le contenu post-launch du jeu.
Kingdom Come : Deliverance II sera disponible le 4 février prochain sur PC, mais aussi sur Xbox Series X|S et PS5. Les fans de la première heure pourront continuer l’aventure de Henry qui prendra place, seulement quelques minutes après la fin des évènements du premier jeu. Ce nouveau périple sera ponctué d’une série de contenus supplémentaires gratuits et payants au cours de l’année 2025 dont voici le détail ci-dessous :
Ces extensions seront accompagnées de plusieurs mises à jour gratuites afin d’améliorer encore davantage l’expérience de jeu Kingdom Come: Deliverance II.
A savoir que vous pourrez obtenir l’expérience complète avec Kingdom Come: Deliverance II Gold Edition, comprenant le jeu de base, le Kit du Chasseur Galant, et le Pass d’Extension. Ce dernier vous donne accès aux magnifiques boucliers du passage des saisons ainsi qu’à trois extensions captivantes. Avec cette édition Gold, c’est tout l’univers du jeu qui s’ouvre à vous.
Cet article Kingdom Come Deliverance 2 recevra du contenu après sa sortie, voici les extensions gratuites et payantes à venir est apparu en premier sur JVFrance.
Activision a confirmé que les joueurs sur console pourront bientôt désactiver le cross-play avec les utilisateurs PC dans le mode de jeu classé de Call of Duty: Black Ops 6 et Warzone.
Face aux demandes répétées des joueurs, Activision a annoncé une nouveauté importante visant à améliorer l’expérience de jeu dans Call of Duty. Dès la Saison 2, qui débutera le 28 janvier, les joueurs sur consoles auront la possibilité de désactiver le cross-play avec les utilisateurs PC dans le mode de jeu classé. Cette fonctionnalité, actuellement en phase de test, s’inscrit « dans l’esprit d’améliorer l’expérience de jeu », a déclaré l’éditeur.
Depuis son lancement en novembre dernier, le mode classé a suscité un engouement massif parmi les joueurs qualifiés (au moins 50 victoires en multijoueur). Cependant, la triche y reste un problème majeur, nuisant à l’équité des matchs. Activision a déjà banni plus de 136 000 comptes suspects, tout en reconnaissant que ce fléau affecte considérablement la communauté.
Pour y remédier, plusieurs mesures ont été mises en place, notamment : De nouvelles détections côté client et serveur pour identifier les comportements suspects, une mise à jour majeure du pilote de niveau noyau pour limiter les triches au niveau système, un système de détection des altérations plus performant. Activision a également précisé pourquoi il n’utilisait pas les interdictions basées sur les adresses IP : Ces méthodes impacteraient injustement des groupes entiers, comme un campus universitaire ou un cybercafé, alors qu’une seule machine est fautive.
En parallèle de ces ajustements, le développeur Treyarch a dévoilé quelques surprises pour les fans du mode Zombies. La Saison 2 introduira une nouvelle carte baptisée The Tomb, promettant des défis inédits pour les amateurs de ce mode coopératif.
Avec ces améliorations et nouveautés, Activision espère répondre aux attentes des joueurs et renforcer l’équilibre dans l’écosystème compétitif de Black Ops 6 et Warzone.
Cet article Le cross-play de Call of Duty: Black Ops 6 et Warzone bientôt désactivable sur consoles est apparu en premier sur JVFrance.
Annoncé le jour de l’anniversaire de sa sortie en Early Access, Palworld dévoile une feuille de route avec d’intéressants ajouts à son contenu. Une annonce chargée en révélations importantes qui risque de faire suer Big N.
Au programme : de l’histoire et des améliorations !
Future Roadmap
We've been updating the game since the start of Early Access, and we'll continue to update Palworld as we prepare for the official release!The future roadmap includes the following:
-CO-OP Crossplay
-World Transfers for Pals
-Final Boss / Ending Scenario… pic.twitter.com/k8UuMhg5r1— Palworld (@Palworld_EN) January 19, 2025
Cette feuille de route se sépare en deux types d’annonces : les mises à jour majeures, comme les nouveaux événements. Et les améliorations du jeu de base. Comme aucune date n’est annoncée sur cette feuille de route, il n’est pas possible de savoir quelles sont les priorités du studio. Mais comme toutes fondations, il est plutôt certain que Pocket Pair prévoit d’améliorer la base de Palworld, qui est encore en Early Access, on rappelle. Et oui, malgré 25 millions de joueurs présents le mois de son lancement et les centaines de milliers encore actif sur Steam, Palworld n’est pas encore un jeu complet. Mais ça ne saurait tarder !
On peut imaginer que l’ensemble de ces mises à jour auront surement lieu courant 2025 et pourront se terminer lors du second anniversaire du jeu, en janvier 2026, afin de marquer le coup. Dans le but de célébrer cet événement avec sa communauté et les fans de Pals, Pocket Pair vous propose de voter pour votre ou vos petites créatures favorites (ou humains favoris ? Bizarre…) Un joli moyen de garder la hype, tout en permettant à chacun de comparer ses choix sur les réseaux et avec le résultat final une fois qu’il sera dévoilé.
Alors que l’on pouvait s’attendre à un enlisement dans une tourmente sans fin pour Pocket Pair et Palworld avec des problèmes juridiques, la licence affiche un état de santé plus que prometteur. De quoi ravir les amateurs de chasses aux créatures fantastiques.
Cet article Palworld fête son anniversaire et révèle ses futures mises à jour : nouveaux Pals, boss de fin et un monde amélioré. est apparu en premier sur JVFrance.